Space As The New Frontier
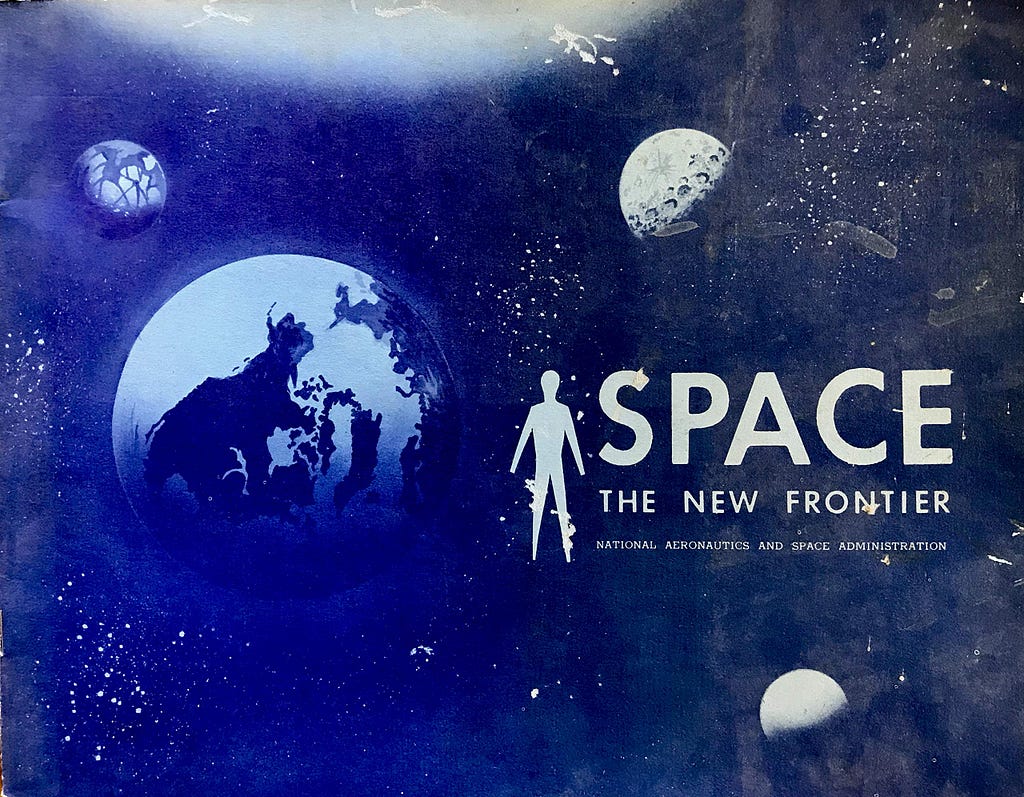
Solar Sail?
- A solar sail, simply put, is a spacecraft propelled by sunlight. Whereas a conventional rocket is propelled by the thrust produced by its internal engine burn, a solar sail is pushed forward simply by light from the Sun. This is possible because light is made up of packets of energy known as “photons,” that act like atomic particles, but with more energy. When a beam of light is pointed at a bright mirror-like surface, its photons reflect right back, just like a ball bouncing off a wall. In the process the photons transmit their momentum to the surface twice — once by the initial impact, and again by reflecting back from it. Ever so slightly, propelled by a steady stream of reflecting photons, the bright surface is pushed forward.
- A solar sail is made up of just such a reflective surface, or several surfaces, depending on the sail’s design. When the bright sails face the Sun directly, they are subjected to a steady barrage of photons that reflect off the shiny surfaces and impel the spacecraft forward, away from the Sun. By changing the angle of the sail relative the Sun it is possible to affect the direction in which the sail is propelled — just as a sailboat changes the angle of its sails to affect its course. It is even possible to direct the spacecraft towards the Sun, rather than away from it, by using the photon’s pressure on the sails to slow down the spacecraft’s speed and bring its orbit closer to the Sun.
- In order for sunlight to provide sufficient pressure to propel a spacecraft forward, a solar sail must capture as much Sunlight as possible. This means that the surface of the sail must be big — very big. Cosmos 1 is a small solar sail intended only for a short mission. Nevertheless, once it spreads its sails even this small spacecraft will be 10 stories tall, as high as the rocket that will launch it. Its eight triangular blades are 15 meters (49 feet) in length, and have a total surface area of 600 square meters (6500 square feet). This is about one and a half times the size of a basketball court.
- For a true exploration mission the requirements are far greater: when a NASA team in the 1970s, headed by Louis Friedman, suggested using a solar sail spacecraft for a rendezvous with Halley’s comet, they proposed a sail with a surface area of 600,000 square meters (6.5 million square feet). This is equivalent to a square of 800 meters (half-mile) by 800 meter — the size of 10 square blocks in New York City!
- Even with such a gigantic surface, a solar sail spacecraft will accelerate very slowly when compared to a conventional rocket. Under optimal conditions, a solar sail on an interplanetary mission would gain only 1 millimeter per second in speed every second it is pushed along by Solar radiation. The Mars Exploration Rovers, by comparison, accelerated by as much as 59 meters (192 feet) per second every second during their launch by conventional Delta II rockets. This acceleration is 59,000 times greater than that of a solar sail!
- But the incomparable advantage of a solar sail is that it accelerates CONSTANTLY. A rocket only burns for a few minutes, before releasing its payload and letting it cruise at a constant speed the rest of the way. A solar sail, in contrast, keeps on accelerating, and can ultimately reach speeds much greater than those of a rocket-launched craft. At an acceleration rate of 1 millimeter per second per second (20 times greater than the expected acceleration for Cosmos 1), a solar sail would increase its speed by approximately 310 kilometers per hour (195 mph) after one day, moving 7500 kilometers (4700 miles) in the process. After 12 days it will have increased its speed 3700 kilometers per hour (2300 mph).
- While these speeds and distances are already substantial for interplanetary travel, they are insignificant when compared to the requirements of a journey to the stars. Given time, however, with small but constant acceleration, a solar sail spacecraft can reach any desired speed. If the acceleration diminishes due to an increasing distance from the Sun, some scientists have proposed pointing powerful laser beams at the spacecraft to propel it forward. Although such a strategy is not practicable with current technology and resources, solar sailing is nevertheless the only known technology that could someday be used for interstellar travel.
Space Gym
- America might not need yet another exercise machine. Treadmills, weights and resistance equipment already jam the health clubs.
- Yet Gravity machines have found a niche at the YMCA in this resort town. On a recent weekday morning, a dozen women and men push, pull and grunt on the machines’ slanted glideboards. They push with their legs, then pull with their arms — up and down diagonally.
- “Keep those legs up!” trainer Yonka Perkins tells her straining students. “Keep those glutes nice and tight!”
- The $17.6 billion health club industry is always open to something new. The YMCA is one of roughly 30 health clubs in the United States to add Gravity machines since the start of the year. It’s not clear if this relatively new device will lead to a hot new trend like Spinning, a popular indoor cycling program, but so far it offers a glimpse into an industry always looking to entice exercisers with new ways to stay tone and trim.
- “We’re always looking for ways to make exercise more effective, more fun, less time, more pop for your time,” said Brooke Correia of the International Health, Racquet and Sportsclub Association.
- Gyms have come a long way since the low-tech days of Indian clubs and medicine balls. Rudimentary machines like the motorized belts that jiggled users’ bellies have given way to sophisticated machines that keep tabs on cardiovascular activity and simulated distance.
- Gravity equipment competes for precious floor space not only with standbys like StairMasters and Pilates equipment, but with newer machines that feature everything from video screens to vibrating bases. Gravity’s hook is that it can offer a range of cardio and strength-training exercises — you can even do Pilates on it — in a short period of time. The track is adjustable; the steeper the incline, the harder the workout.
- “It’s very intense,” said Joanne Leiser after a 30-minute workout at the Saratoga YMCA. “It’s quick and it’s cool.”
- The machines were introduced in 2003 by San Diego-based efi Sports Medicine, creator of the Total Gym. Jesse Campanaro, national sales manager, said sales have been brisk recently, climbing from 130 clubs to more than 160 in the United States since the start of the year.
- These are small numbers compared to the estimated 12.65 million people who used resistance machines in 2005, or the 10.5 million who ran on treadmills. But as Campanaro put it, “We’re getting a lot more traction.”
- The machines are essentially a health club version of the company’s less expensive Total Gym, a home and rehabilitation machine known to many for the TV infomercials featuring martial arts actor Chuck Norris confidently gliding up and down slantwise.
- No infomercials for Gravity, though the system has clearly benefited from the enthusiasm of health club fitness trainers. Jon D’Alessio, group fitness director at The Jungle Club in Vero Beach, Fla., said it’s a fast, efficient way to provide both personal training or group sessions. To keep the interest of men, he developed exercises that simulate golf swings and kayak paddling.
- “It’s much more fun than whatever else I’ve been doing for the last 15 years,” he said.
- The machines are often given studio space for group classes of up to a dozen.
- The Saratoga YMCA placed its machines in a semicircle inside the hairpin bend of the indoor track, where Perkins regularly leads group sessions there to the beats of Beyonce and Shakira. The 30-minute “total body strengthening” classes have been so popular she has had to add sessions. People show up at 5:30 a.m. to sign up for a 6 a.m. class.
- “It’s not beating the heck out of your body,” said student Lori Tynan. “The machine keeps everything smooth.”
Space Robots
- Applications outside the Earth’s atmosphere are clearly a good fit for robots. It is dangerous for humans to get to space, to be in space and to return from space. Keeping robots operating reliably in space presents some unique challenges for engineers. The ultra-high vacuum in space prevents the use of most types of lubricants. The temperatures can swing wildly depending on whether the robot is in the sun light or shade. And, or course, there is almost no gravity. This is actually more of an opportunity than a challenge and leads to the possibility of some unique designs. The conceptual robot at left has 21 independent joints. On earth it would be impossible for this robot to support its own weight, but in space, the design presents some unique capabilities. The robot can reach around obstacles and through port holes. The robot also possesses a huge degree of fault tolerance. It can continue to operate with excellent dexterity even after several joints fail.
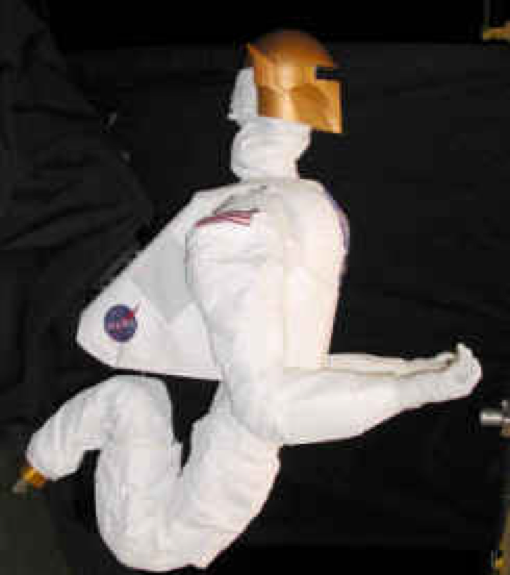
- This robot at is called Robonaut. It is a humanoid robot designed by the Robot Systems Technology Branch at NASA’s JSC in a collaborative effort with DARPA. Robonaut’s creators designed it to have dexterity, range of motion and task capabilities roughly equivalent to that of an astronaut in a space suit. Space flight hardware has been designed for servicing by astronauts for the last fifty years. It makes sense that robots would gradually pick up these tasks over time rather than suddenly replacing astronauts. The set of tools used by astronauts during space walks was the initial design consideration for the system. This drove the development of Robonaut’s dexterous five-fingered hand and human-scale arm. The robot’s mix of sensors includes thermal, position, tactile, force and torque , with over 150 sensors per arm. The control system for Robonaut includes an onboard CPU with miniature data acquisition and power management in an environmentally hardened body. He’s also got a nifty thermal suit to protect him from the wild temperature swings in space.
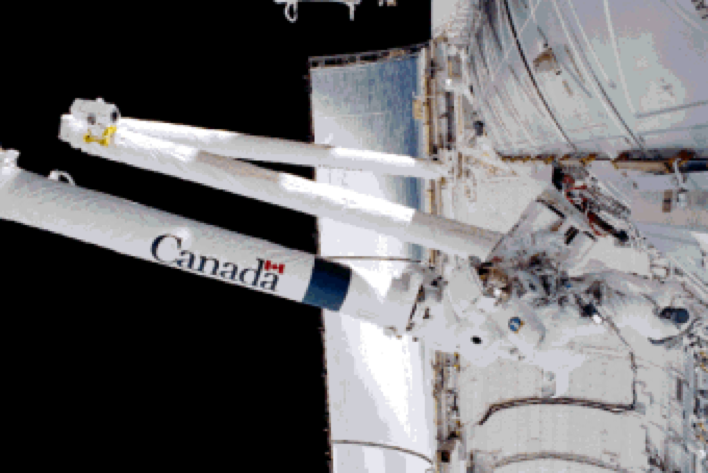
Here we see the Canadarm robot arm, a version of which has flown on every Space Shuttle flight for the last twenty years. The arm has a shoulder with 2 DOF, an elbow with 1 DOF and a 3 DOF wrist. The arm is routinely used as a mobile work platform for the astronauts, for “tossing” satellites into space and for retrieving faulty ones. Non-routine uses have included: knocking a block of ice from a clogged waste-water vent, pushing a faulty antenna into place, and activating a satellite that failed to go into proper orbit. Several of these arms have been in service for twenty years. A true robot success.
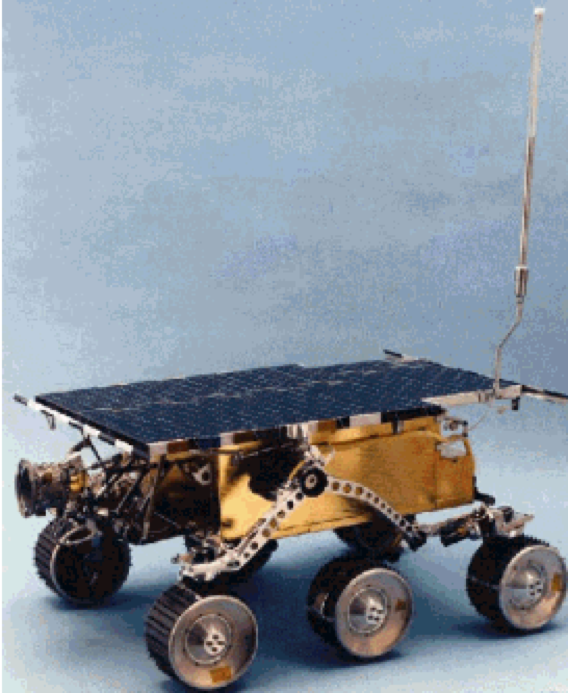
- Here we see a press photograph of the Sojourner mobile robot that ultimately explored the surface of Mars. This is more of an R/C car than a robot as it was completely remote controlled from Earth, but NASA calls it a robot so I will too. In any case, the pictures it provided from the Martian surface were breath taking. Sometimes I think that really cool pictures may be NASA’s greatest contributions. The deep field images produced by the Hubble telescope are in my opinion some of the greatest wonders of mankind.
- The Sojourner is a 6-wheeled vehicle of a rocker bogie design which allows the traverse of obstacles a wheel diameter (13cm) in size. Each wheel is independently actuated and geared (2000:1). The front and rear wheels are independently steerable, providing the capability for the vehicle to turn in place. The vehicle has a top speed of 0.4m/min. It is powered by a 0.22sqm solar panel comprised of 13 strings of 18, 5.5mil GaAs cells each. The normal driving power requirement for the microrover is 10W.
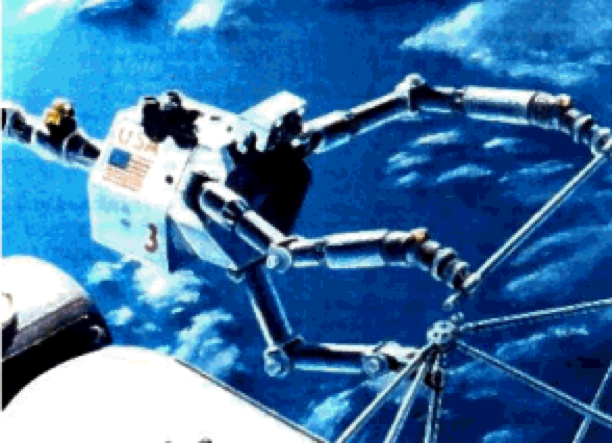
- NASA decided to develop a $288-million Flight Telerobotics Servicer (FTS) in 1987 to help astronauts assemble the Space Station, which was growing bigger and more complex with each redesign. Shown here is the winning robot design by Martin Marietta, who received a $297-million contract in May 1989 to develop a vehicle by 1993. About the best thing that can be said for the FTS project was that it generated a lot of lessons learned. The robot never flew and never will fly because it was never completed. This project demonstrated that fault-tolerance gone wild will doom a robot. The robot had so many redundant systems that there was just too much to go wrong.
Space Inhabitation
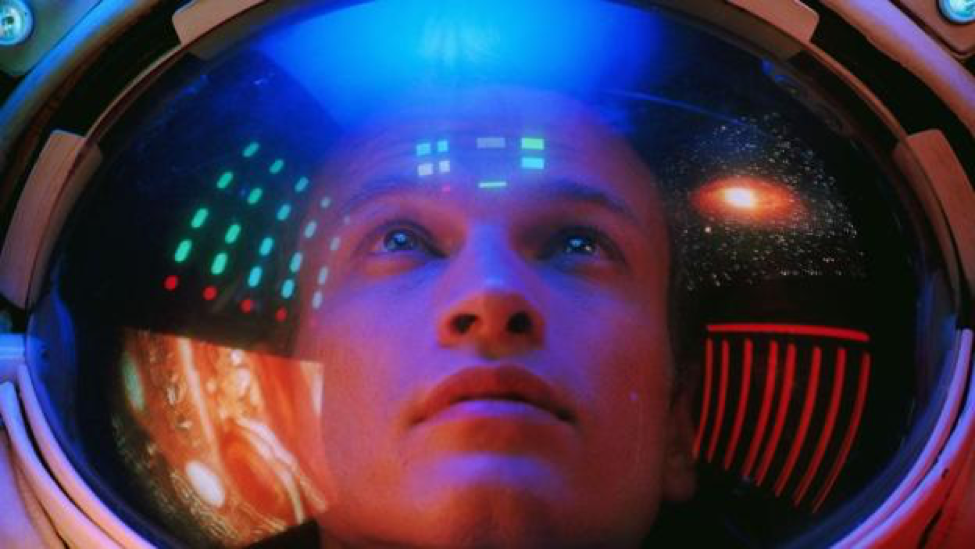
Why should we take the idea of colonising space seriously?
- With our rising planet’s population competing for space and resources, some people are convinced we need to look beyond Earth to help ensure humanity’s survival. As Elon Musk, the entrepreneur behind space tourism company SpaceX put it recently: “I think there is a strong argument for making life multi-planetary in order to safeguard the existence of humanity in the event that something catastrophic were to happen.”
- Even if you don’t believe this bleak vision, it’s hard to ignore the eternal human instinct to discover the undiscovered — an urge that could push people beyond the safety of our planet. And there might not be as many hurdles as you might think. “We are at the level of technology where we can imagine leaving the planet for a few nearby places in our Solar System,” former astronaut Jeffrey Hoffman, who will present his ideas at our summit, told BBC Future previously. “The Moon is just around the corner, and Mars isn’t that far away. We have the possibility of at least making the first steps of those voyages in our own lifetimes.”
What could a space colony look like?
- One possible idea goes as far back as the 1920s. Austro-Hungarian rocket pioneer Herman Potočnik imagined a circular spacecraft — rather like a flying saucer — that rotates to create artificial gravity while a large, concave mirror could focus sunlight for an energy source. As far-fetched as this may sound, the idea has lingered over the years — most notably in the mid-1970s by the late Princeton physicist Gerard O’Neill, and again by the space think tank British Interplanetary Society. Before you dismiss the idea of floating colonies completely, it’s worth noting that the British Interplanetary Society predicted we would reach the Moon three decades before it actually happened.
What about life on Mars? Or any other planet?
- Other experts favour the idea of setting up home on firmer ground — a planet or moon — creating an artificial “biosphere” with all the necessary elements for human life to thrive. Mars has become a main focus of attention, and astonishingly, people are already trying to make steps to set-up a new civilisation there by 2025. The Dutch project, called Mars One, was launched in 2012 and it has already vetted 40 applicants from a pool of 200,000. They should now receive training as part of a reality TV show that is helping to fund the project. Needless to say, Mars One has its detractors — but it does at least show the vast interest in colonising space.
- SpaceX’s Musk is also rumoured to have his sights set on populating the Red Planet with a colossal vehicle called the Mars Colonial Transporter, which if it were reusable could cut the costs of return trips. As he told Aeon recently, that would only be the beginning: “If we can establish a Mars colony, we can almost certainly colonise the whole Solar System, because we’ll have created a strong economic forcing function for the improvement of space travel. We’ll go to the moons of Jupiter, at least some of the outer ones for sure, and probably Titan on Saturn, and the asteroids.” Even Musk draws the line at inhabiting other stars, however. “Alpha Centauri is four light years away, so if you go at 10% of the speed of light, it’s going to take you 40 years, and that’s assuming you can instantly reach that speed, which isn’t going to be the case… I just wonder what humanity will even look like when we try to do that.”
OK, forget the stars. But how would we cope with life in space? Or getting there?
- Life on the International Space Station reveals some of the massive challenges we would face. Simply ferrying enough water for its six inhabitants costs around $2 billion per year. That’s not to mention the difficulties of providing food and oxygen. Ideally, a space colony would need to become self-sufficient, generating all these resources itself or perhaps mining them from nearby asteroids.
- Then there are the stresses to the human body: reduced gravity can lead to bone and muscle loss and a dangerous build-up of pressure in the head, which can sometimes lead to temporary and persistent eye problems. Cosmic radiation, meanwhile, can cause cataracts and increase the risk of cancer. Sleep loss and loneliness, meanwhile, could wreak havoc on the mind. Any space colony would need to solve all these difficulties, and more — including surprising day-to-day practical issues, such as the fact that some materials — including human facial hair — tend to be more flammable in lower gravity.
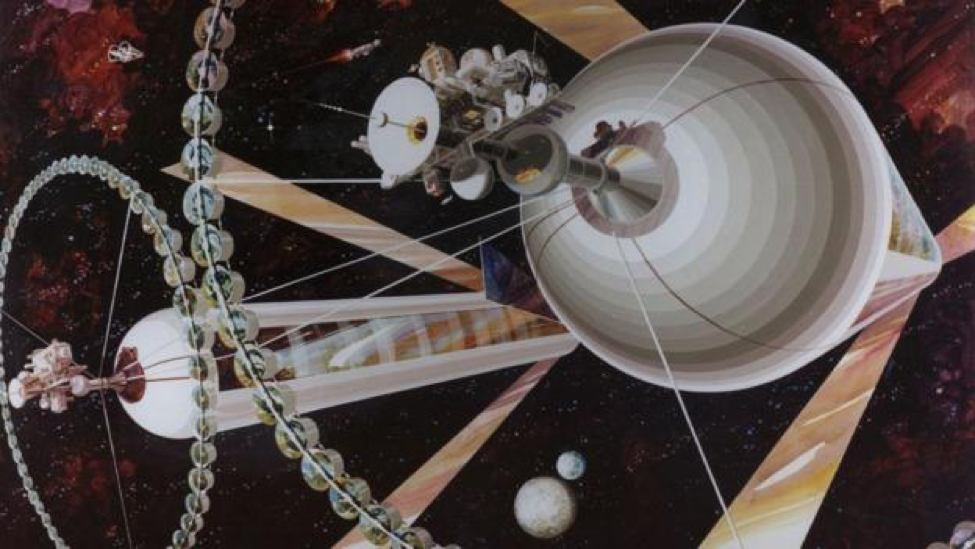
- Even more flammable could be our relationship with our fellow companions. With the aim of simulating the long journey to Mars, researchers on the Mars500 project locked up six willing participants in a small sealed hatch in Moscow — with living quarters less than 80 metres squared — for 520 days. One participant’s sleep cycle became skewed so that he was rarely around the rest of the “crew”. Another developed signs of depression while a third started to suffer from cognitive impairment.
- And that’s not to mention the political issues of how these isolated human beings should be governed and how to prevent strife in these fragile new societies. While that may seem a distant problem, some scientists and philosophers have already started to draw up a “bill of rights” for these future civilisations.
Let’s say we succeed. Would people living or born in space be different from you or I?
- Provided humans can reproduce in space — and given astronauts’ known health problems, that’s by no means certain — these isolated colonies would soon take on unique cultures. They would probably develop their own languages, and may even evolve new physical characteristics. According to Cameron Smith of Portland State University, an initial space colony of 2,000 individuals would begin to look and act differently within about 300 years — with mutations giving rise to different hair texture, skin type and stature — replacing our stocky bodies with something that’s easier to manoeuvre in low gravity. Smith even speculates that a future population may turn to genetic engineering to design radical new organs — such as protection against cosmic rays and gill like structures that would allow them to draw oxygen from carbon dioxide — which might could allow Martians to venture out of the artificial biosphere and truly inhabit their new home.
Space Elevator
- HOW THE SPACE ELEVATOR COULD OPEN UP INTERPLANETARY SUPPLY CHAINS.
- The prohibitive cost of lifting payloads out of the Earth’s atmosphere is hamstringing humanity’s conquest of the solar system. The space elevator may soon make chemical rockets a thing of the past.
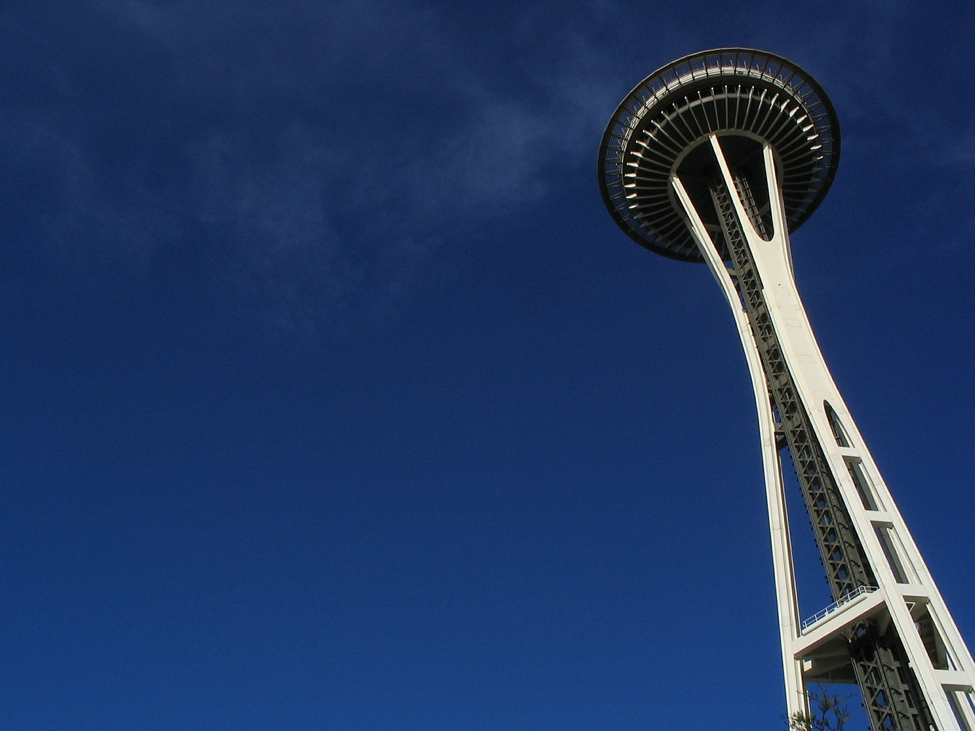
- At the Coupa Inspire conference in May this year, keynote speaker Richard Branson announced plans to have Virgin Hotels orbiting the planet within 40 years.
- Branson’s famous “anything is possible” attitude was on display, as he breezily talked of shuttle trips between his space hotels and the surface of the moon, and observatory domes where guests can marvel at the Earth from above.
- Branson’s audience predominantly consisted of procurement professionals, many of whom were turning their minds to the challenge of maintaining a supply chain in space.
- Considering the vast amount of goods and services that flow through any mere terrestrial hotel, the prospect of supplying a space hotel, or any other off-planet settlement, is daunting.
The Payload Challenge
- It’s unbelievably expensive to send cargo into space. These days, all eyes are on SpaceX. Elon Musk’s company is leading the way in reducing the cost of payload delivery through lean operations, integrated engine production and reusable spacecraft.
- At full capacity, the Falcon 9 rocket can lift cargo to low-earth orbit at US$1233 per pound ($2719 per kg). NASA is paying SpaceX $133 million per mission to resupply the International Space Station. This equates to $27,000 per pound ($59,500 per kg) of cargo delivered.
- Reducing the cost of payload delivery is one of the highest priorities for Musk, who has stated that $500 per pound ($1100 per kg) or less is an achievable goal.
- Even with payload cost being driven ever-lower, the expense still makes the prospect of a regular delivery service (such as a space hotel supply chain) prohibitively expensive.
- Tech Insider recently published a playful article working out the hair-raising costs of some of the unnecessary items NASA has launched into space. They calculated that astronaut Kjell Lindgren’s bagpipes, for example, would have cost anywhere from $54,600 to $259,000 to deliver.
- The International Space Station’s espresso machine weighs 44 pounds (20kg), and would have cost between $400,400 and $1.9 million to deliver.
The Space Elevator — A Better Way to Lift Cargo into Space
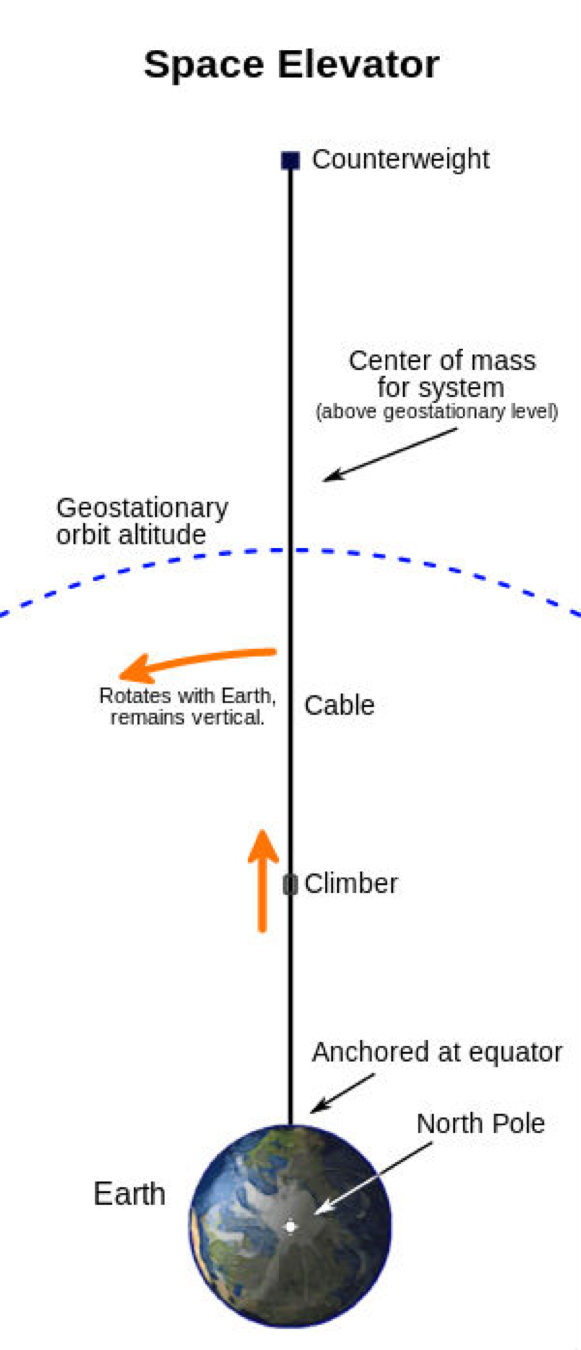
- Arthur C. Clarke predicted that the space elevator would be built “about 10 years after everyone stops laughing”. That’s because at first glance, it seems like pure science-fiction. The thing to understand about how the space elevator would work is that it isn’t a tower or ladder to space, but rather a tether.
- The Earth-end of the tether would be attached to the surface near the equator, while the other end would be anchored to an object in space (most likely a space-station) beyond geostationary orbit, or 35,800km in altitude. The tether would therefore be held stationary under tension as the space station tried to “pull away” from the planet.
- At present, no material exists with the tensile properties required to construct the tether, but teams all over the world are working on the challenge.
- Recently, carbon nanotubes, boron nitride nanotubes, and diamond nanothreads have all been considered viable new materials, enabling scientists to inch ever closer to the required tensile strength.
- There are many other challenges involved, but commentators agree that once the tether question has been solved, the other components of the elevator will be relatively simple to design and construct.
A Freight Train to Space
- Once constructed, laser or solar-powered ‘climbers’ would ascend and descend the tether, taking materials and passengers to geostationary orbit. Payload prices could be as low as $100 per pound ($220 per kg), with two added advantages.
- Firstly, proponents predict a working elevator would be significantly safer than chemical rocket technology. And secondly, the climbers would operate continuously.
- Journalists often write about the space elevator in the singular, but there is no reason why the planet would only have one. In fact, it’s likely that multiple competitive nations (and private enterprises) would insist on having their own.
Opening Up Space
- With working space elevators, the enormous expenditure of fuel used in boosting chemical step-rockets up through our atmosphere will become a thing of the past.
- Spacecraft will no longer be needed for surface-to-space lifts or descents. Instead they will only be needed to move from point to point in space. After an initial boost, a craft in space simply falls freely along its trajectory, with only short-term adjustments and deceleration required.
- Space elevators need not be limited to Earth. Within the next century, we may “drop” shorter tethers to the surface of the moon and Mars, with regular cargo and passenger services plying their way between the space stations at the top of the elevators. The complex task of keeping a Moon or Mars colony supplied would become much more feasible.
- But that’s thinking a long way ahead. In the medium-term future, Branson’s luxury space hotel may well sit atop a space elevator, supplying its every need.
- In the short-term, any day now we may read that scientists have discovered materials strong enough to construct the tether. At which point — as Arthur C. Clarke predicted — everyone will stop laughing.
Skyhooks, Space Elevators and Space Exploration
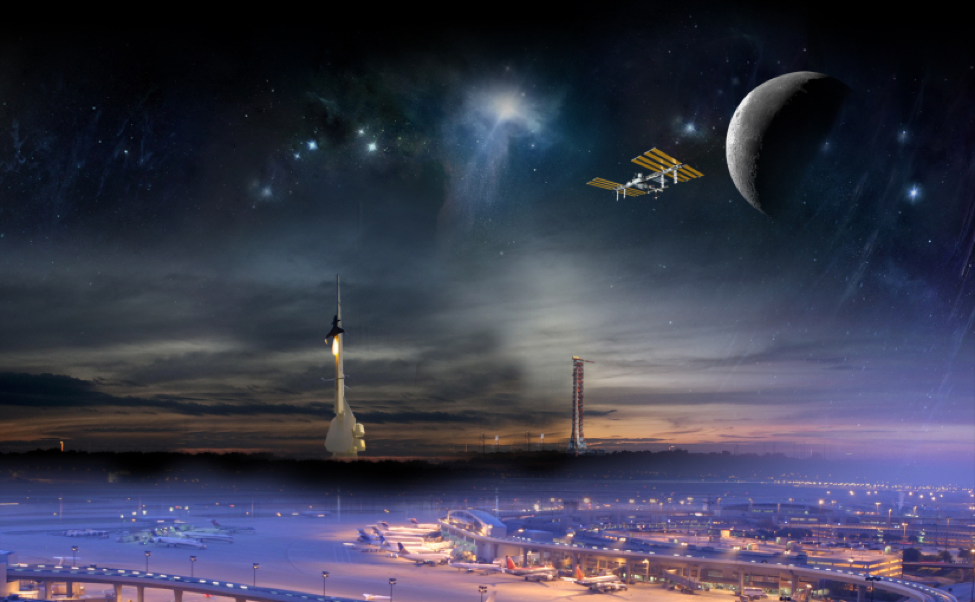
- It is about the ideas and technologies that will enable the affordable movement of people and goods from the surface of the Earth to orbit and beyond. It is about the ideas and technologies that will allow mankind to explore, develop, and colonize space.
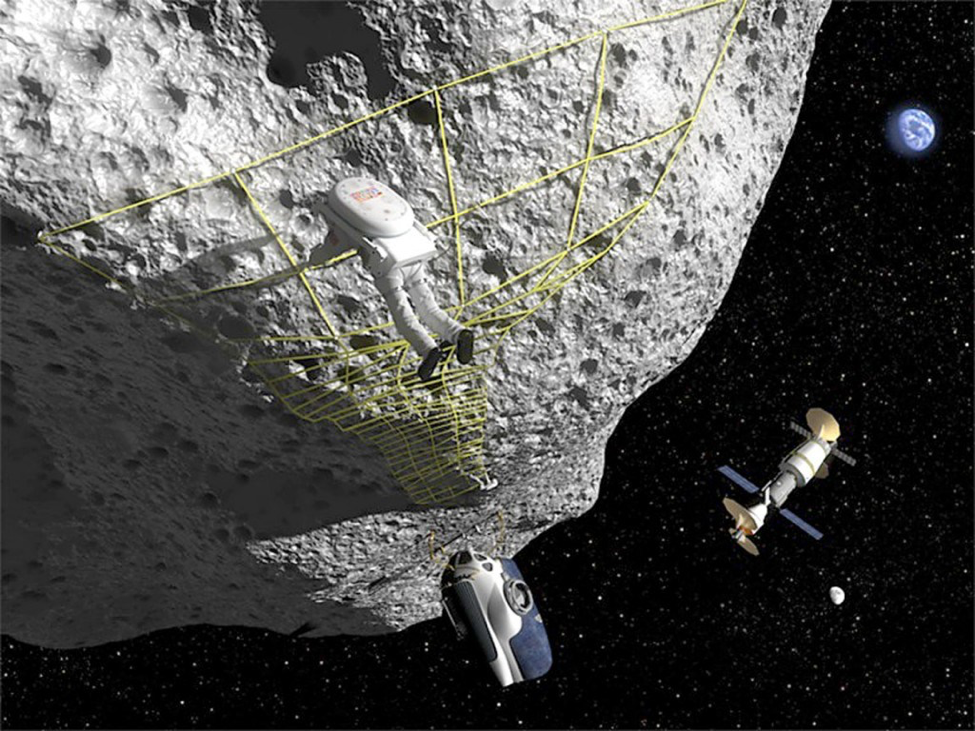
_______________________________________________________
1: Skyhooks and Space Elevators
refers to a group of three proposed space transportation concepts that their promoters claim will make Earth-to-orbit and interplanetary spaceflight affordable, thereby opening the way for the commercial development of lunar mining, asteroid mining, space-based solar powerstations, space colonies, and colonies on the Moon, Mars, and in the asteroids.
These three concepts are:
- A non-rotating Earth surface to geostationary orbit Space Elevatoras described by Arthur C. Clarke in his book The Fountains of Paradise.
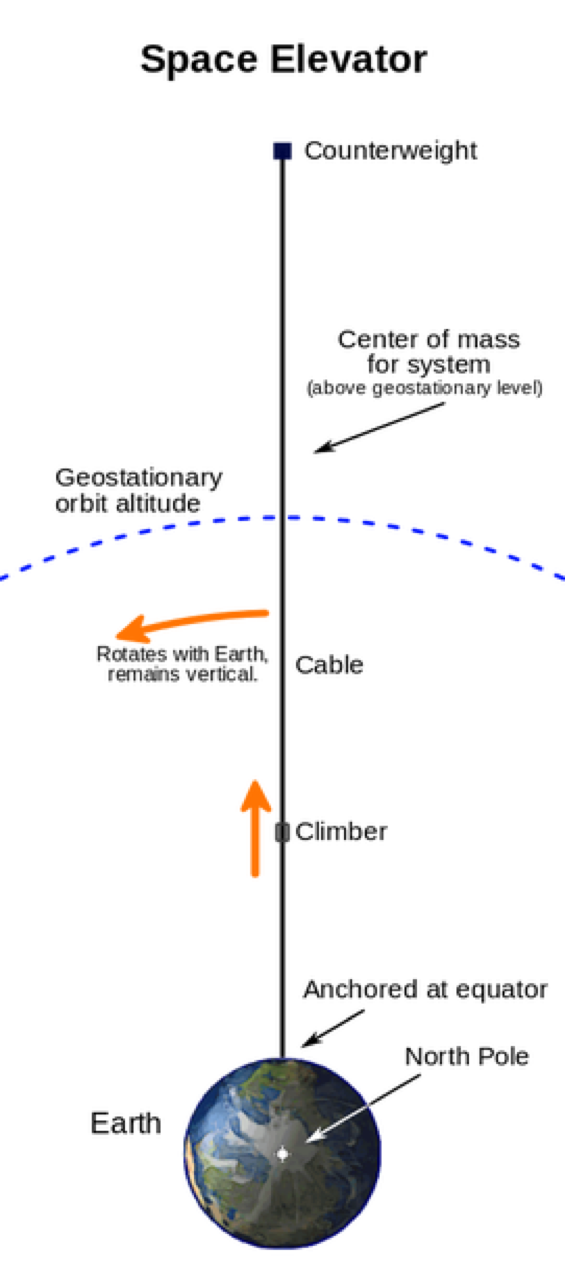
_______________________________________________________
2) A non-rotating Skyhook for Earth as first proposed by E. Sarmont in 1990.
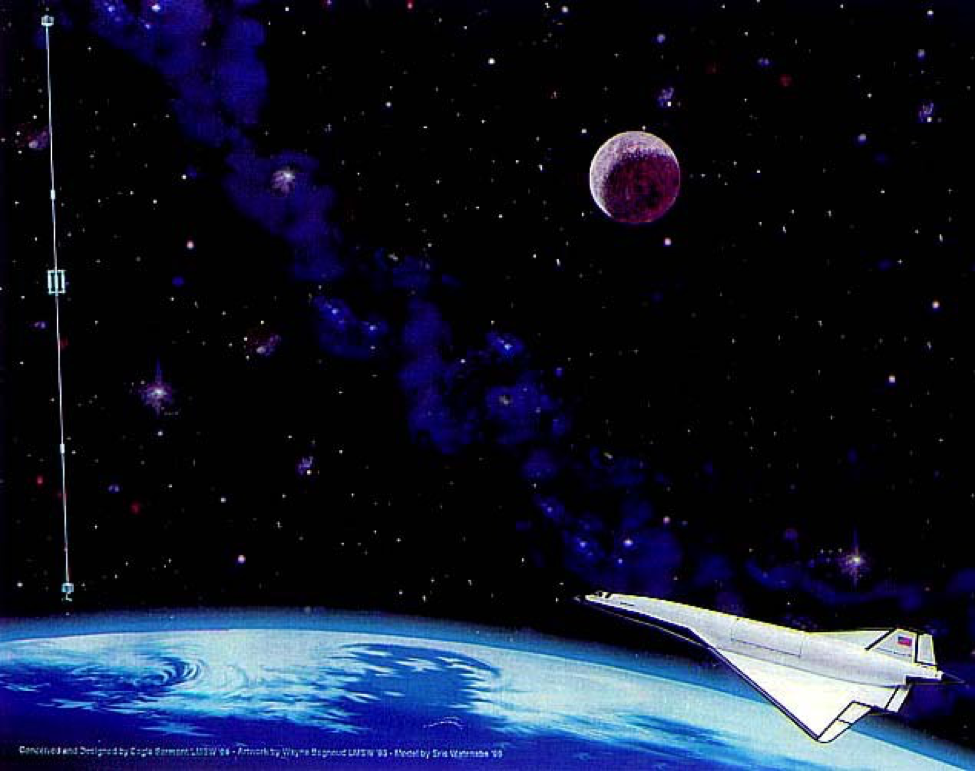
_____________________________________________________
3) A rotating Skyhook as first proposed by H. Moravec in 1977.
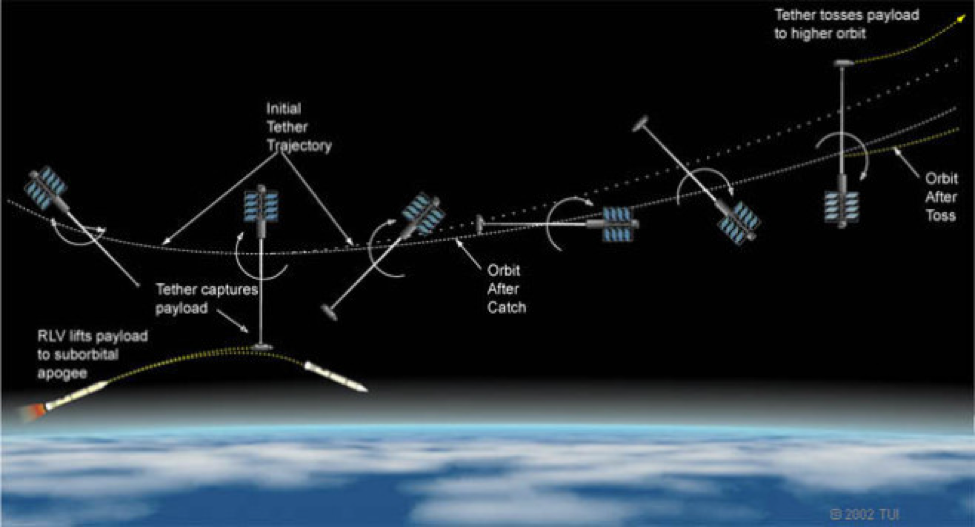
_____________________________________________________
All three of these concepts have advantages and disadvantages. The most significant of them being:
- the rotating and non-rotating Skyhooks can be built with currently existing materials and technology while the Earth surface to geostationary orbit Space Elevator cannot,
- the rotating and non-rotating Skyhooks require a suborbital launch vehicle to get the lower end of the tether while the Earth surface to geostationary orbit Space Elevator does not,
- the rotating and non-rotating Skyhooks require either an electrodynamic or ion propulsion system for altitude control while the Earth surface to geostationary orbit Space Elevator does not.
Of these three items the most important to remember is that both the rotating and non-rotating Skyhooks can be built with existing materials and technology while the Earth surface to geostationary orbit Space Elevator cannot.
- The following is a direct quote from “Space Elevators, An Advanced Earth-Space Infrastructure for the New Millennium”, a NASA Conference Paper that discusses all three of these concepts.
“The LEO space elevator [non-rotating Skyhook] is an intermediate version of the Earth surface to GEO space elevator concept, and appears to be feasible today using existing high-strength materials and space technology.”
___________________________________
- The following is a direct quote from “Hypersonic Airplane Space Tether Orbital Launch System” (HASTOL), a NASA funded study on rotating Skyhooks.
“The fundamental conclusion of the Phase I HASTOL study effort is that the concept is technically feasible. We have evaluated a number of alternate system configurations that will allow hypersonic air-breathing vehicle technologies to be combined with orbiting, spinning space tether technologies to provide a method of moving payloads from the surface of the Earth into Earth orbit. For more than one HASTOL architecture concept, we have developed a design solution using existing, or near-term technologies. We expect that a number of the other HASTOL architecture concepts will prove similarly technically feasible when subjected to detailed design studies. The systems are completely reusable and have the potential of drastically reducing the cost of Earth-to-orbit space access.”
____________________________________
- The following is a quote from Keith Henson, co-founder of the L-5 Society regarding the Earth surface to geostationary orbit Space Elevator.
“No current material exists with sufficiently high tensile strength and sufficiently low density out of which we could construct the cable. There’s nothing in sight that’s strong enough to do it — not even carbon nanotubes.”
____________________________________
- While no Skyhook capable of capturing an arriving spacecraft has been built so far, there have been a number of flight experiments exploring various aspects of the Skyhook/space tether concept.
_________________________________________________________
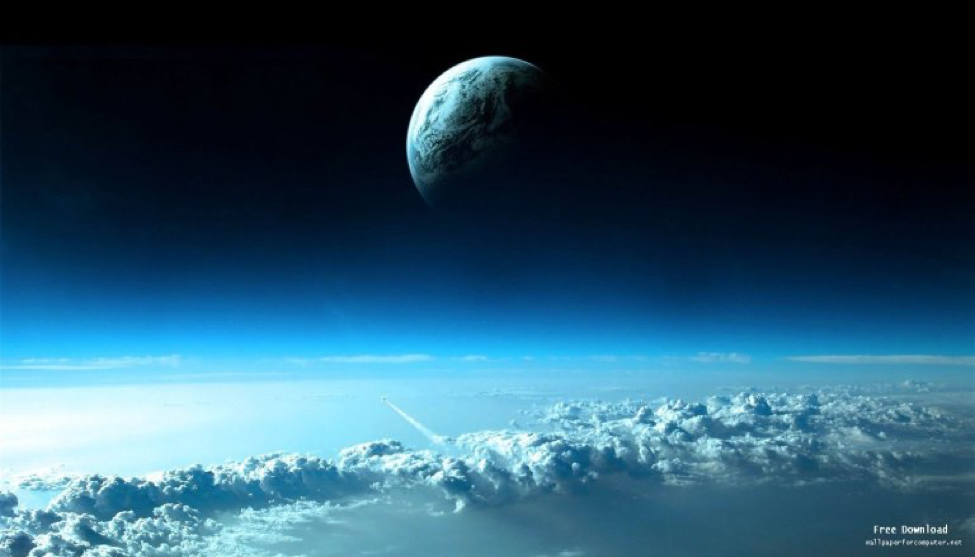
2: Affordable spaceflight and Skyhooks
The three most important issues that need to be addressed if a transportation system is to be commercially affordable on a mass-market level are:
- reusability,
- low propellant fraction,
- high payload fraction.
Reusability so the investment can be amortized over a large number of trips, a low propellant fraction for reduced operating costs, and a large payload fraction so that the cost of each trip can be distributed over a large amount of payload. Trains, planes, automobiles, trucks, and ships are all very good examples of this. Currently existing expendable rockets are not.
- When a launch vehicle takes-off to go to Earth orbit, approximately 90% of its take-off weight is propellant, 9% is the weight of the rocket, and 1% is the weight of the payload.
- Compared to trains, planes, automobiles, trucks, and ships, it should be fairly obvious why the currently existing expendable launch vehicles are somewhat of a failure when it comes to meeting the three main requirements for a commercially affordable mass-market transportation system.
The reasons for this failure are threefold:
- the speed the rocket needs to achieve to reach Earth orbit,
- the amount of propellant it takes to achieve that speed (which has to do with the performance of the rocket motor),
- the lack of reusability of the launch vehicle.
The total speed that a launch vehicle needs to achieve in order to reach low Earth orbit is approximately 9,400 m/s. This includes the speed of low Earth orbit, plus additional velocity for overcoming the force of gravity that is trying to pull the rocket down, and additional velocity for the atmospheric drag as the launch vehicle climbs through the atmosphere.
- A Skyhook makes spaceflight affordable by reducing the speed the rocket needs to achieve in order to reach low Earth orbit. That reduction in speed means less propellant is required. The reduction in propellant allows the size of the payload to be increased. A larger payload and less propellant reduces the cost of getting to orbit.
In order to provide some order of magnitude examples of how different sized reductions in velocity translate into increased payload capacity, some simple calculations were made using the ideal rocket equation, the 90% propellant, 9% rocket, and 1% payload ratio, and the 9,400 m/s total speed for orbit value. The results of these calculations show that:
- reducing the speed required for orbit by 200 m/s increases the payload size by 50% and reduces the cost by 33%,
- reducing the speed required for orbit by 400 m/s doubles the size of the payload and reduces the cost by 50%,
- reducing the speed required for orbit by 800 m/s triples the size of the payload and reduces the cost by 67%,
- reducing the speed required for orbit by 1,600 m/s increases the payload by a factor of 6 and reduces the cost by 84%.
This process continues as the Skyhook gets longer and applies to both rotating and non-rotating Skyhooks.
- Reusability of the launch vehicle is the third key issue that needs to be addressed if a Skyhook space transportation system is to become commercially affordable on a mass-market level. Using some of the increased payload capacity that the Skyhook makes possible to make the launch vehicle reusable is an idea that is used and discussed in every reference in this article that discusses either rotating or non-rotating Skyhooks. The specific amount of extra weight that will be required to make a launch vehicle reusable will depend on the details of the launch vehicle design. Is it single-stage-to-orbit, two-stage-to-orbit, ground launched, air launched, catapult launched, air-breathing, all rocket, or a combination propulsion system? All of these will have different requirements in order to make the launch vehicle reusable. But regardless of the details, using some of the increased payload capacity made possible by the Skyhook to go from an expendable launch vehicle to a reusable launch vehicle, will also be a very important step in the process of making spaceflight mass-market affordable: which is the whole purpose of a Skyhook.
________________________________________________________
3: Non-rotating Skyhooks
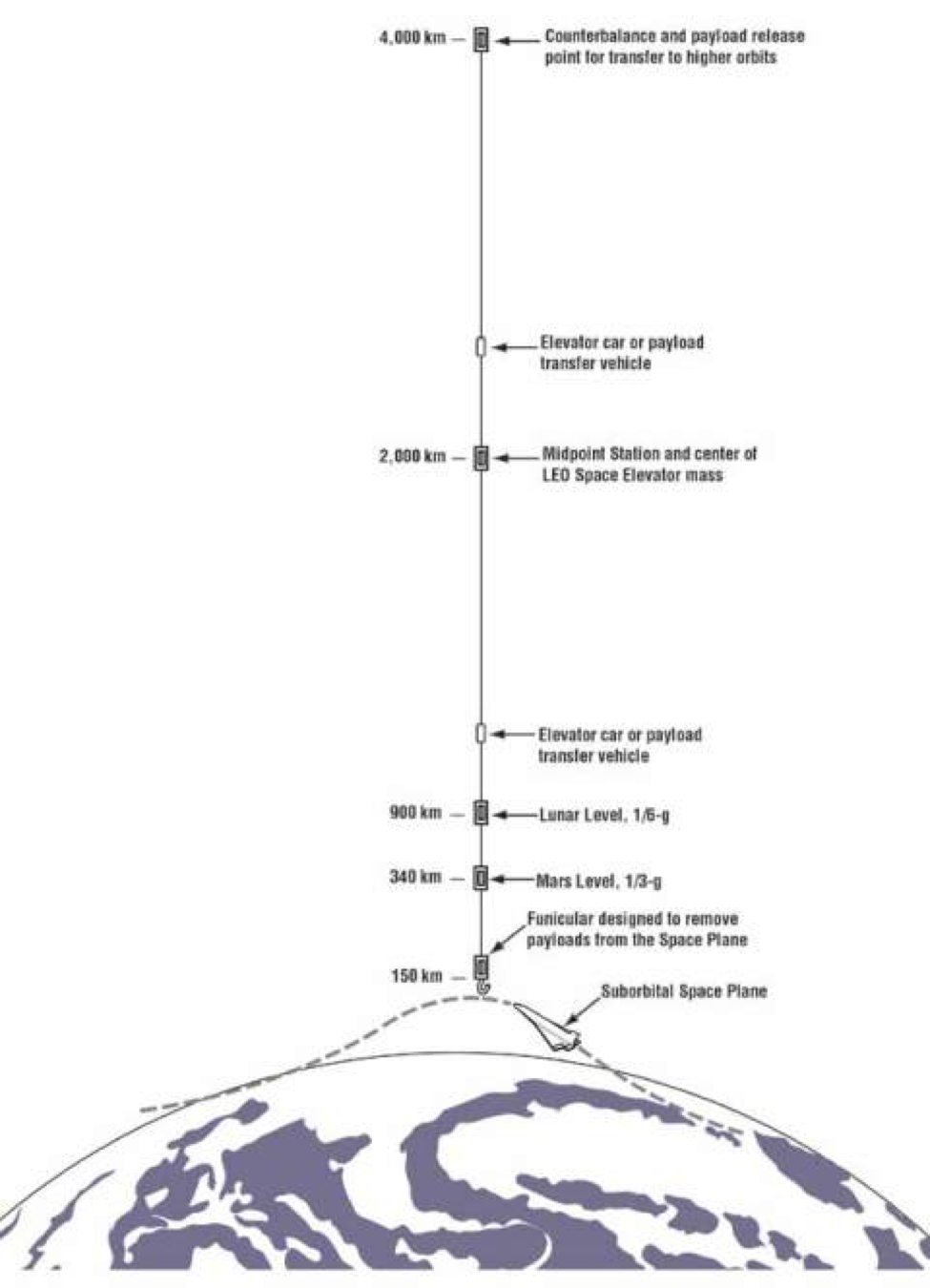
- A non-rotating Skyhook is a vertically oriented, gravity gradient stabilized tether whose lower endpoint does not reach the surface of the planet it is orbiting. As a result the lower end of the cable appears to be hanging from the sky, hence the name skyhook. The idea of using a tidally stabilized tether for downward-looking Earth observation satellites was first proposed by the Italian scientist Giuseppe Colombo.
- The idea of using a non-rotating Skyhook as part of a space transportation system for Earth where sub-orbital launch vehicles would fly to the bottom end of the tether, and spacecraft bound for higher orbit, or returning from higher orbit, would use the upper end of the tether, was first proposed by E. Sarmont in 1990, expanded on in a second paper in 1994, and in a third paper in 2014.
- Other scientists and engineers, as well as NASA, Lockheed Martin, former astronaut Bruce McCandless II, and Dr. Robert Zubrin, have also investigated, validated, and added to this concept.
- In addition, NASA representatives who have reviewed this concept have described it as, “The first idea we have seen that offers a believable path to $100 per pound to orbit.”
Conceptually the main difference between a non-rotating Skyhook and an Earth surface to geostationary orbit Space Elevator is that the non-rotating Skyhook does not reach down to the surface of the Earth. The lower end of the non-rotating Skyhook is above the upper edge of the atmosphere and requires a high-speed aircraft/sub-orbital launch vehicle to get there. Since the lower end of the Skyhook is moving at less than orbital velocity for its altitude, a launch vehicle flying there can carry a larger payload than it could carry to orbit on its own. When the cable is long enough, Single-Stage To Skyhook flight with a reusable sub-orbital launch vehicle becomes possible. In addition, unlike a Space Elevator that remains over the same spot on the Earth, a non-rotating Skyhook circles the planet every few hours. This allows the Skyhook to serve as a terminal for sub-orbital launch vehicles arriving from just about anywhere on Earth. This type of Skyhook can start out as short as 200 km and grow to over 4,000 km in length using a bootstrap method that takes advantage of the reduction in launch costs that come with each increase in tether length.
- At its longest, a mature non-rotating Skyhook is approximately 1/25th the length of the 100,000 km long Space Elevator. As a result, it is much lighter in mass, and can be affordably built with existing commercially available carbon fiber materials. Analysis has also shown that this saving in construction cost for the non-rotating Skyhook more than makes up for the additional cost of the sub-orbital launch vehicle that it requires. As a result, a mature non-rotating Skyhook with reusable single stage sub-orbital launch vehicle is considered to be cost competitive with what is thought to be realistically achievable using a Space Elevator, assuming a Space Elevator can ever be built.
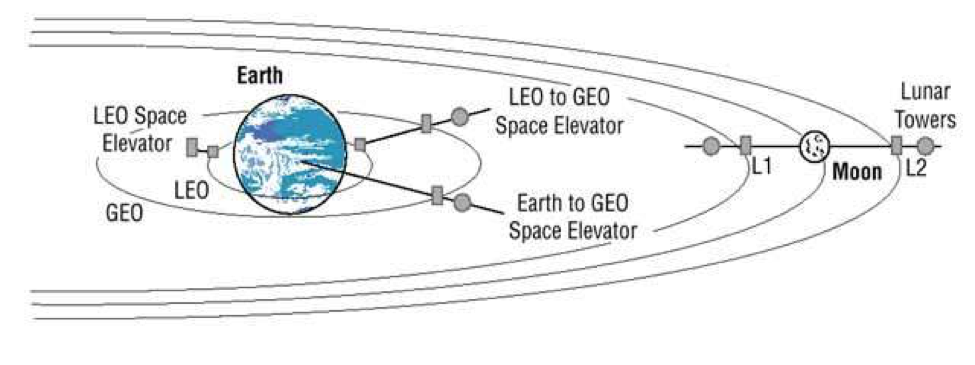
- A visual comparison of the various Space Elevator and non-rotating Skyhook concepts that have been proposed (the non-rotating Skyhook is referred to as a LEO Space Elevator in this drawing)
_____________________________________________
- Another advantage of the non-rotating Skyhook is that once it is long enough, the upper end of the cable will be moving at just short of escape velocity for its altitude. This means that a spacecraft such as the Orion spacecraft could be placed on either a free-return orbit to the Moon, or on course for a near-Earth asteroid, without the need for an expensive expendable upper stage for boosting it to escape velocity. Elimination of the expendable upper stage and all the propellant it will require will also dramatically reduce the number of flights to the lower end of the non-rotating Skyhook, which will further reduce the cost of such a mission.
- This ability to capture sub-orbital launch vehicles coming up from the Earth at the lower end of the cable, and to launch spacecraft to higher orbits from the upper end of the cable, requires energy. Energy that comes from either a solar powered ion propulsion system or an electrodynamic propulsion system on the Skyhook. The advantage of this over current launch systems is the greatly improved operating efficiency and reduced cost of either of these propulsion systems compared to conventional expendable rockets.
- While these high-efficiency, low-thrust propulsion systems cannot be used for a planetary surface to orbit launch system due to their low thrust, they are perfect for use on an orbiting Skyhook due to their ability to gradually store up energy by raising the orbital altitude of the Skyhook between arriving and departing flights.
_________________________________________________
The Docking Maneuver
- The process of docking an arriving spacecraft at the lower end of the non-rotating Skyhook starts with the Skyhook in an elliptical orbit. The low point of this elliptical orbit, the perigee, is selected so that the lower end of the Skyhook cable will be at an altitude of 185 km at that point in the orbit. The altitude of the high point of the elliptical orbit, the apogee, is selected based on the mass of the arriving spacecraft.
- In addition to boosting the arriving spacecraft to the proper speed and altitude for rendezvous, the sub-orbital launch vehicle for this flight will also need to time its take-off so that the arriving spacecraft will rendezvous with the lower end of the Skyhook when the Skyhook is at the low point of its orbit.
- When the arriving spacecraft docks with the lower end of the Skyhook it will lower the center of gravity of the total Skyhook system, thereby pulling the Skyhook down into a lower more circular orbit. If the apogee altitude of the Skyhook’s initial elliptical orbit was properly selected, the Skyhook will end up in a circular orbit after the arriving spacecraft has docked.
- Upon completion of the docking maneuver, the Skyhook’s solar-powered ion propulsion system, or electrodynamic propulsion system, is activated so as to start raising the orbital altitude of the Skyhook back to its original altitude.
- What makes the Skyhook concept work is energy exchange. When the Skyhook is in its initial elliptical orbit it is in a higher-energy orbit than the one it ends up in after the arriving spacecraft docks with the lower end of the Skyhook. What happens is that the Skyhook gives the extra energy of the higher elliptical orbit to the arriving sub-orbital spacecraft; a spacecraft that doesn’t have enough energy to remain in orbit on its own. The end result is that the arriving spacecraft gets an energy boost from the Skyhook that allows it to remain in orbit while the Skyhook gives up energy and drops to a lower energy orbit. Before another arriving spacecraft can dock at the lower end of the Skyhook, the Skyhook will need to use its high efficiency low thrust propulsion system to raise itself back to the original higher-energy orbit.
______________________________________________
4: An Initial Non-rotating Skyhook
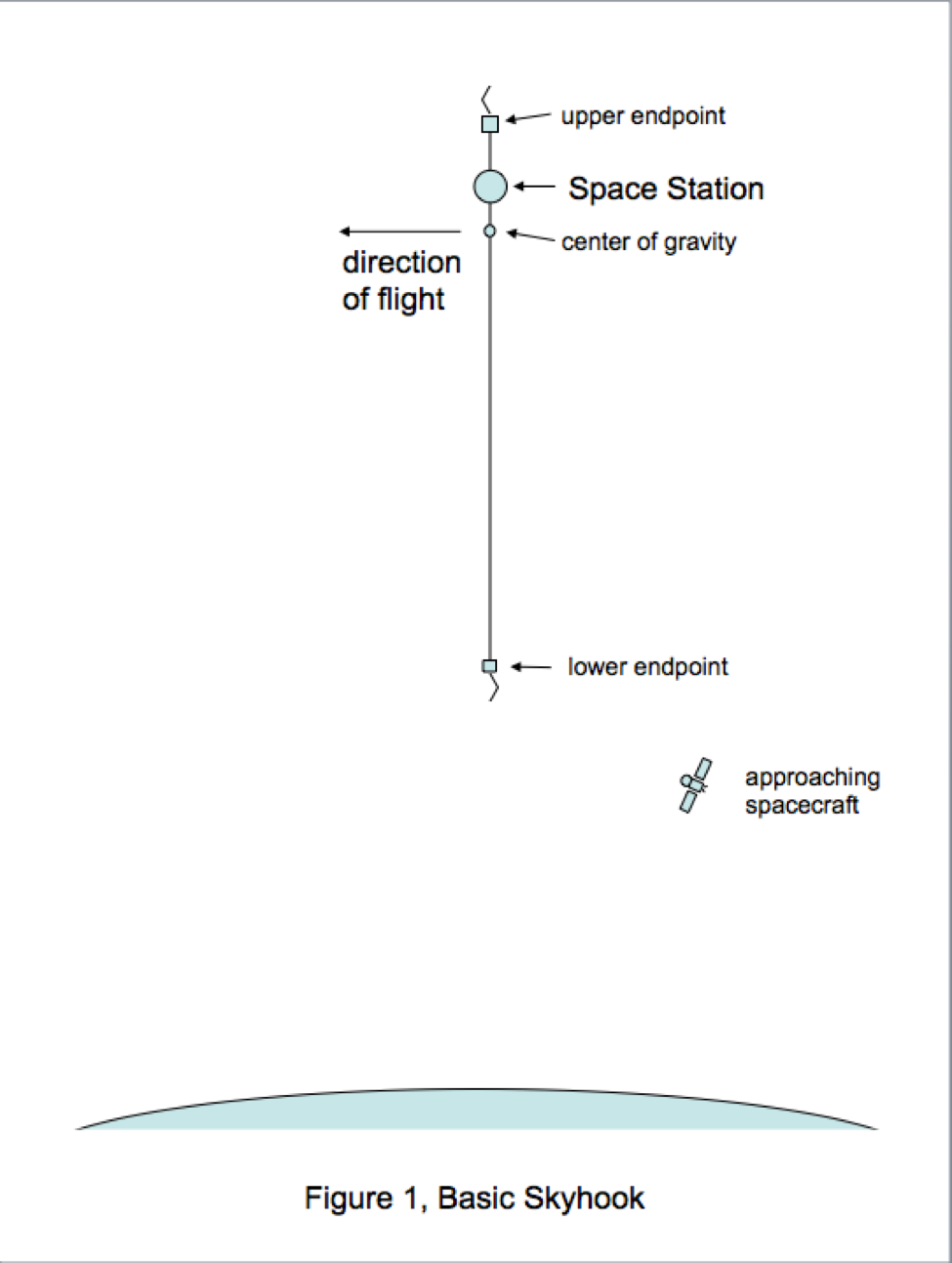
- Assume a 200 km long 5,600 kg tether with a 2,000 kg docking crane at each end, a 100,000 kg space station, and a 40,000 kg ion propulsion/power system designed for a flight rate of 12 flights per year. The baseline orbit for this basic start-up Skyhook is an elliptical orbit that has a low point altitude of 350.6 km and a high point altitude of 445.3 km. As with all Skyhooks, it is the center of gravity of the system that is in orbit. Since the speed required for orbit decreases with altitude, those parts of the Skyhook that are below the center of gravity are moving at less than orbital velocity for their altitude and want to move closer to the Earth, while those parts that are above the center of gravity are moving faster than orbital velocity for their altitude and therefore want to move further away from the Earth. The end result is a vertically oriented tether that is under constant tension. Due to this tension, the tether/space station combination acts like a long thin tower with a weight that can be positioned anywhere along its length and the center of gravity of the system is determined by the vertical location of the space station on the tether.
- Note: the mass for the tether used in this example was calculated using the fiber properties for the T1000GB carbon fiber as shown on the TORAY Industries website, a thermoplastic matrix, a 65% fiber volume, a safety factor of 5 to 1, and an arriving spacecraft mass of 14,800 kg.
- Preparing for an Arriving Spacecraft In order to prepare the Skyhook for docking with a spacecraft that is coming up from the Earth, the space station will need to be positioned 170.3 km up from the bottom end of the tether. This will put the center of gravity of the Skyhook at the 165.6 km mark. This means that when the Skyhook system is at the low point of its baseline orbit, the bottom end of the tether will be 185 km above the surface of the Earth moving at 96.67% of circular orbit velocity for that altitude.
- The Launch System The launch system used for this example is a launch vehicle and spacecraft similar in size and performance to the Falcon 9 launch vehicle and Dragon spacecraft. This particular launch vehicle and spacecraft combination has the ability of delivering a 6,000 kg useful payload to a 185 km high circular due east orbit. The calculated useful payload for this system when flying to a 335 km high circular due east orbit is 5514 kg. The calculated useful payload for this system when flying to the bottom of this Skyhook is 7,736 kg. That represents a 40% increase in useful payload when flying to the Skyhook compared to flying directly to a space station in a 335 km high orbit that does not have a Skyhook. Assuming the same cost per flight both with and without the Skyhook, this basic Skyhook will reduce the launch cost of this launch system by 29% compared to flying direct to a space station without a Skyhook. This reduction in launch cost will continue to improve as the length of the Skyhook increases.
- Hooking Up When an arriving spacecraft docks with the bottom of the Skyhook it lowers the center of gravity of the system. If the arriving spacecraft has a mass of 14,800 kg, it would lower the center of gravity of the Skyhook by 15.6 km: from 165.6 km above the bottom of the tether down to 150 km. This also changes the orbit of the Skyhook from the initial 350.6 x 445.3 km high elliptical orbit to a 335 km high circular orbit.
- Bringing the Spacecraft and Space Station Together Once the arriving spacecraft is firmly attached to the bottom end of the tether, the space station starts pulling the tether up. At the same time this also pulls the space station down closer to the Earth. The end result is that the center of gravity of the Skyhook stays in the same 335 km high circular orbit while the space station and spacecraft gradually come together and dock.
- The Orbit Raising Maneuver While the space station is gradually moving down the tether towards the spacecraft, the Skyhook’s ion propulsion system is activated in order to start raising the orbital altitude of the Skyhook. Once the arriving spacecraft has docked with the space station and the crew change has occurred, the spacecraft is lowered ~45 km down from the space station. Since the departing spacecraft is moving at less than orbital velocity while hanging on the tether at this altitude, no retro rocket burn is required for the return to Earth, and the release of the spacecraft will need to occur when the Skyhook is in the proper position over the Earth for the departing spacecraft to arrive at its planned landing site. Prior to the release of the departing spacecraft, the docking crane at the lower end of the Skyhook will be used to salvage the spacecraft’s solar array/trunk module in order to add it to the Skyhook’s power system. This solar array is normally discarded and allowed to burn up in the atmosphere when the spacecraft is departing from a space station that is not equipped with a Skyhook. After the departing spacecraft has gone, the ion propulsion system will continue thrusting until the Skyhook is back in its baseline 350.6 x 445.3 km elliptical orbit in time for the next arriving spacecraft. The ion propulsion system will consume a total of 86.7 kg of propellant for this orbit raising maneuver.
_______________________________________________________
5: The Upper End of a Mature Non-rotating Skyhook
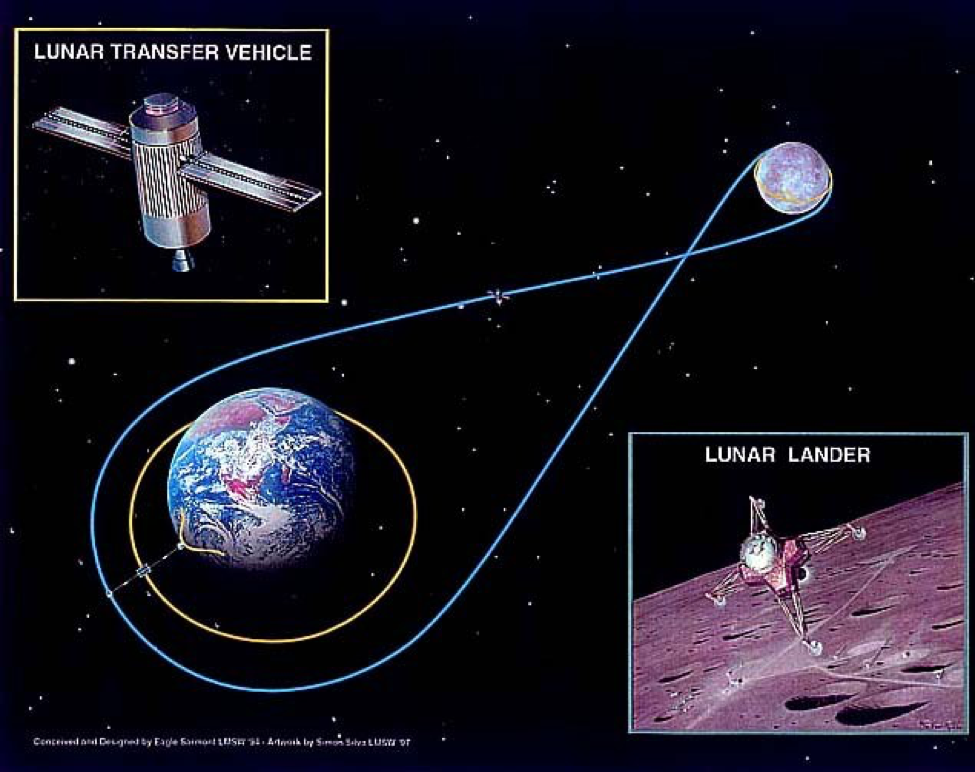
- The upper end of a mature non-rotating Skyhook is designed to be moving at slightly less than escape velocity for its altitude. What this means is that a spacecraft departing from the upper end of the Skyhook can, with the use of only a very small amount of onboard propellant, be headed for geosynchronous orbit, on a free return trajectory to the Moon, on its way to one of the Lagrange Points, or headed for a Near Earth Asteroid.
- To put this in perspective, consider the Saturn V rocket that was used to fly to the Moon in the late ’60s and early ’70s. The Saturn V had a take-off weight of 6,200,000 pounds. Its payload to low Earth orbit was 260,000 pounds. Its useful payload to Earth escape velocity was 100,000 pounds. Everything else, 6,100,000 pounds worth, was used up and thrown away. Now compare this to a mature non-rotating Skyhook launch system.
- A passenger leaves Earth on a highly reusable suborbital spaceplane that takes off from an airport and flies to the bottom end of the non-rotating Skyhook. The passenger transfers from the spaceplane to the Skyhook and the spaceplane returns to Earth with a load of returning passengers and cargo.
- The next step in the journey is a ride on the elevator up the tether to the space station in the middle of the Skyhook where a transfer is made to another elevator that is bound for the upper endpoint. Nothing is thrown away everything is reusable.
- At the upper endpoint station the passenger transfers to a small reusable Earth-Moon spacecraft that is similar to the Apollo Command/Service Module, which will take him/her to lunar orbit. The Earth-Moon spacecraft will use a very small amount of onboard rocket propellant when it departs the upper end of the Skyhook, and will then fire its rocket motor a second time to slow down to lunar orbit velocity when it arrives at the Moon.
- Upon arrival in lunar orbit, the Earth-Moon spacecraft will rendezvous and dock with a reusable single stage Lunar Lander that is carrying passengers and cargo bound for Earth plus additional rocket propellant for the Earth-Moon spacecraft. After the passenger, cargo, and propellant exchange is complete, the Lunar Lander leaves lunar orbit and descends to the surface of the Moon.
- Again, everything is reusable, nothing is thrown away, and the rocket propellant for the Lunar Lander and the Earth-Moon spacecraft comes from the Moon.
______________________________________________________
6: The Earth-Moon Spacecraft
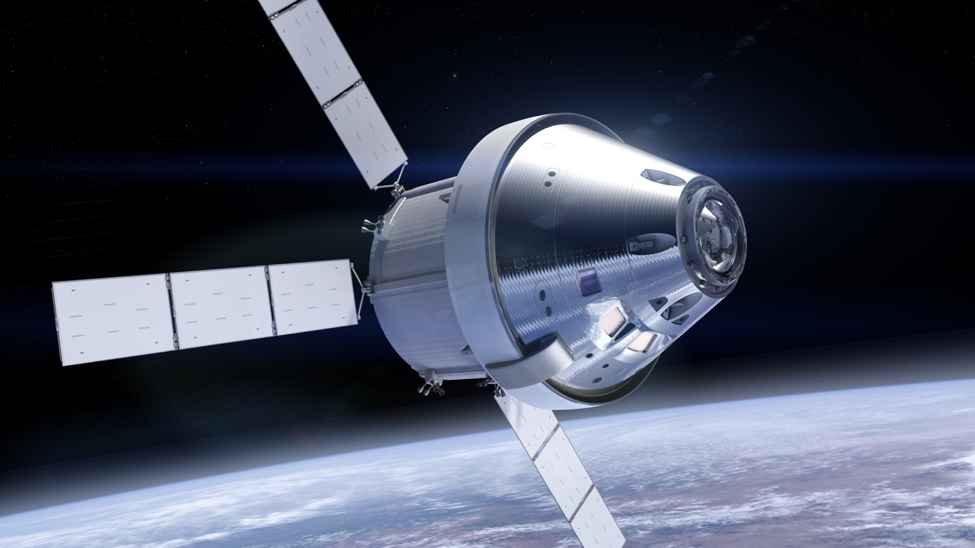
- NASA is currently funding the development of a reusable Earth-Moon/Earth-Asteroid spacecraft that is similar to an Apollo Command/Service Module called the Orion spacecraft. Operate this spacecraft from the upper end of a non-rotating Skyhook and we will have an affordable transportation system for traveling to lunar orbit, the Earth/Moon Lagrange points, and the near Earth asteroids. Add a single stage reusable Lunar Lander and we will have an affordable transportation system for going to the lunar surface. With access to Lunar and/or near Earth asteroid materials, the building of a large, well shielded, rotating, Mars cycler spacecraft becomes possible, and safe regular affordable interplanetary spaceflight to Mars, the Moons of Mars, as well as Ceres and all the other asteroids between Mars and Jupiter, becomes possible.
_______________________________________________________
7: A Non-rotating Skyhook Transportation System for Mars
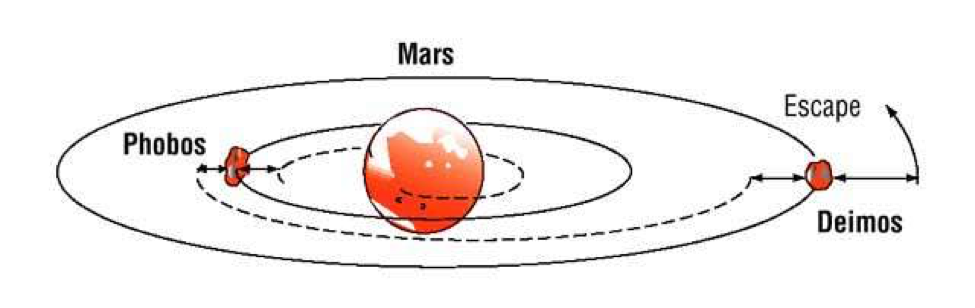
- In 1984, Paul Penzo of JPL proposed a planetary surface to escape velocity tether transportation system for Mars that consists of two unpowered non-rotating Skyhooks; one attached to the Martian moon Phobos, and the other attached to the Martian moon Deimos.
- With this system, a spacecraft arriving at Mars, either direct from Earth, from an outpost space station, or from an Earth-Mars cyclerspacecraft as it swings by Mars, docks at the upper end of the non-rotating Skyhook attached to the outer moon Deimos. The people and cargo on that spacecraft then transfer to an elevator on the Skyhook that will take them down to the lower end of the Deimos Skyhook. There they board a small orbital transfer vehicle that will take them to the upper end of the non-rotating Skyhook that is attached to the inner moon Phobos. Again they transfer to an elevator that will take them to the lower end of the Phobos Skyhook where they will transfer to the reusable single stage Mars Lander that will carry them to the Martian surface. Passengers and cargo from the Martian surface that are bound for either the asteroids, or for Earth, would ride the system in reverse.
- One of the advantages of this concept is that neither Skyhook will require a propulsion system for orbital re-boost or for orbit control, as they both will use the Martian moon they are attached to as a momentum bank to make up any differences in the upward versus downward mass flow of people and cargo. This elimination of the propulsion systems for the two Skyhooks also makes for a significant reduction in the cost to build, and the cost to operate. Like the non-rotating Skyhook for Earth, this two-stage non-rotating Skyhook system for Mars can be affordably built with existing materials and technology.
Space As New Frontier was originally published in Exponential Technologies / Industrial Revolutions Based Infrastructure on Medium, where people are continuing the conversation by highlighting and responding to this story.